About mCDR
Efforts to mitigate the impacts of climate change are gaining interest both in the United States and internationally. Federal agencies, academic institutions, industry, nonprofits and others are researching ocean-based climate mitigation techniques such as marine carbon dioxide removal (mCDR). Marine CDR techniques may have the potential to alleviate certain impacts of climate change by directly or indirectly removing carbon dioxide from the atmosphere and storing it for long periods of time in the ocean.
As a relatively new field of research, there is uncertainty about the efficacy and potential environmental impacts of mCDR techniques. Proposed mCDR activities should be carefully evaluated to avoid unintended and significant adverse impacts to human health or the marine environment.
On this page:
- What Is mCDR?
- Overview of mCDR Techniques
What Is mCDR?
Marine carbon dioxide removal (mCDR) is any ocean-based process or technique designed to remove carbon dioxide from the atmosphere and store it for long periods of time in the ocean. Examples of mCDR techniques include adding alkaline materials to the ocean to increase the amount of carbon stored in ocean waters; adding iron or other nutrients to ocean waters to increase phytoplankton growth and export of carbon to the deep ocean; and sinking organic materials, such as kelp or crop residue, into the deep ocean.
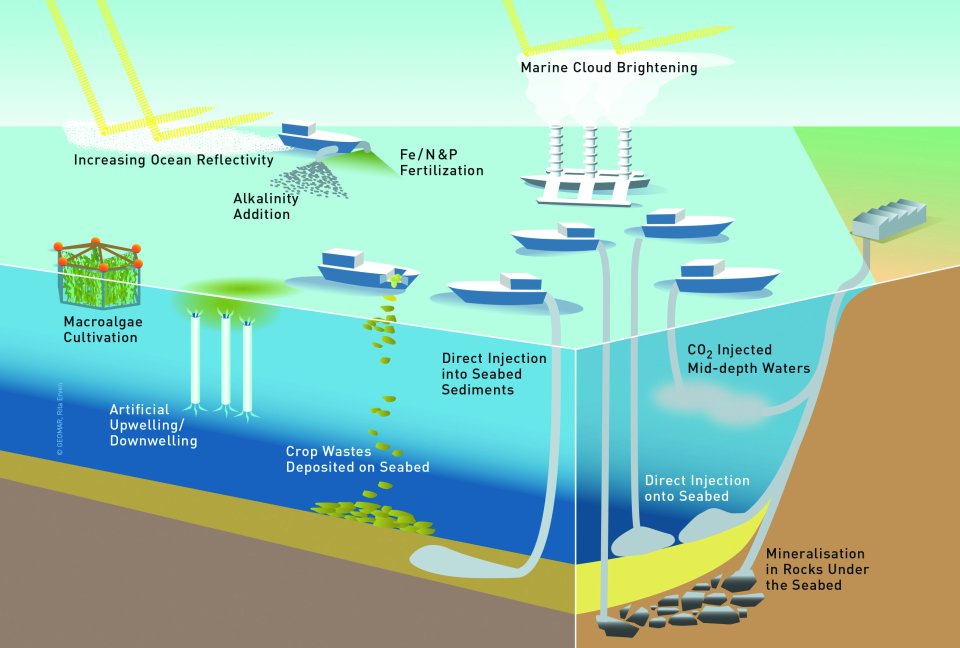
Ocean Alkalinity Enhancement
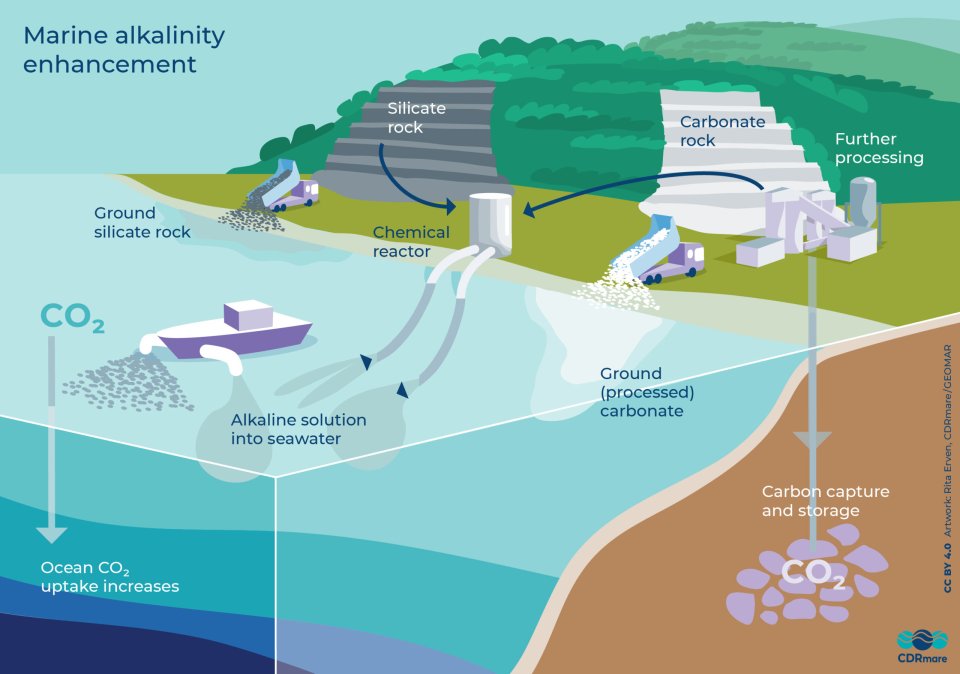
Ocean alkalinity enhancement (OAE) refers to a variety of approaches that aim to increase the alkalinity in ocean water. OAE may be done for the purpose of removing carbon dioxide from the atmosphere and storing it for long periods of time in seawater, primarily in the form of bicarbonate ions, which are a major component of alkalinity in ocean water, or other uses, such as enhancing fisheries production. OAE may also provide the co-benefit of locally mitigating ocean acidification.
Several different methods of OAE are being explored, including:
- Adding alkaline and/or basic solutions directly into seawater.
- Some proposed approaches involve removing acid components from seawater using engineered processes. For example, seawater can be processed using electrochemical or other engineered methods to split seawater into its acid and basic components. The basic component can then be returned to the marine environment, while the acid biproduct is removed and possibly used for other purposes.
- Adding certain types of mined alkaline minerals, such as olivine, to coastal and ocean waters. Under certain oceanographic conditions, alkaline minerals can dissolve into seawater and increase alkalinity.
For OAE to result in a removal of atmospheric carbon dioxide, several processes would need to occur:
- The addition of alkalinity (or removal of acid from seawater) must lead to a net increase in the concentration of alkaline ions in surface seawater. For solid alkaline materials, this would include the dissolution of the solid material in the seawater into its chemical components. Adding alkalinity to seawater (or removing acid from seawater) would lead to a temporary elevation in seawater pH.
- The increase in seawater alkalinity must then result in a series of chemical reactions that convert dissolved carbon dioxide (already present in the seawater) into dissolved inorganic carbon, primarily in the form of bicarbonate. Bicarbonate is one of the most abundant components of seawater and is a relatively stable form of carbon.
- To result in carbon dioxide removal, the seawater (now depleted in dissolved carbon dioxide) must then take up additional carbon dioxide from the atmosphere at the ocean surface and store the dissolved inorganic carbon on climate-relevant timescales. This movement of atmospheric carbon dioxide from the air to the ocean can only occur when the carbon dioxide depleted water is interacting with the atmosphere at the surface of the ocean.
Potential marine environmental impacts of this approach include:
- Toxic effects on marine organisms from exposure to trace metals contained in alkaline minerals or from interacting with mineral particulates in the water.
- Changes in habitat characteristics, ocean biogeochemical cycling, or ecological communities due to localized peaks in seawater alkalinity or pH.
-
Biogeochemical effects from the introduction of additional nutrients such as silicate or iron.
Ocean Fertilization
Marine plants such as phytoplankton (e.g., algae) use carbon dioxide from their environment during photosynthesis. Ocean fertilization activities aim to increase the growth of phytoplankton in the ocean (i.e., enhance ocean primary productivity) for the purpose of increasing carbon export to the deep ocean or other uses, such as enhancing fishery productivity. Ocean fertilization does not include conventional aquaculture, mariculture or the creation of artificial reefs.
Increasing phytoplankton growth could be accomplished by adding nutrients, such as iron, to the open ocean to create a phytoplankton bloom.
Nutrients for ocean fertilization could be added in the form of a liquid or solid material, or nutrients may be moved from the deep ocean to the surface ocean through a process called "artificial upwelling."
For ocean fertilization to result in a removal of atmospheric carbon dioxide, three processes would need to occur:
- Ocean fertilization must lead to increased growth of phytoplankton, which consolidates carbon and nutrients together into organic material.
- Organic material from the phytoplankton bloom must be transferred into the deep ocean so that it does not break down near the surface, releasing its captured carbon back to the atmosphere.
- This transfer of carbon from the surface ocean to the deep ocean must result in a subsequent transfer of carbon from the atmosphere into the surface ocean.
Potential marine environmental impacts of this approach include:
- Growth of toxin-producing algae (i.e., harmful algal blooms).
- Deep ocean deoxygenation from biomass decomposition.
- Decreases in ocean productivity in adjacent areas of the ocean, also known as "nutrient robbing."
- Increases in oceanic production of greenhouse gases (methane, nitrous oxide).
- Changes to ecosystem structure and function.
- Acidification of the deep ocean.
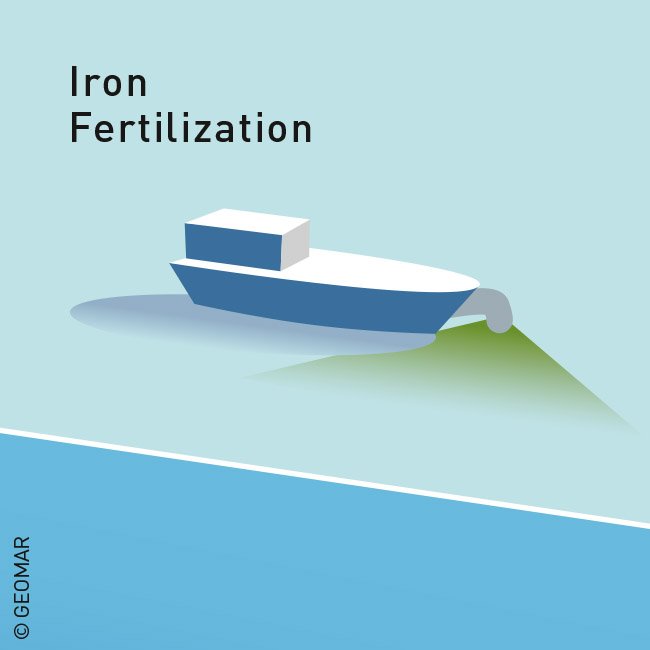
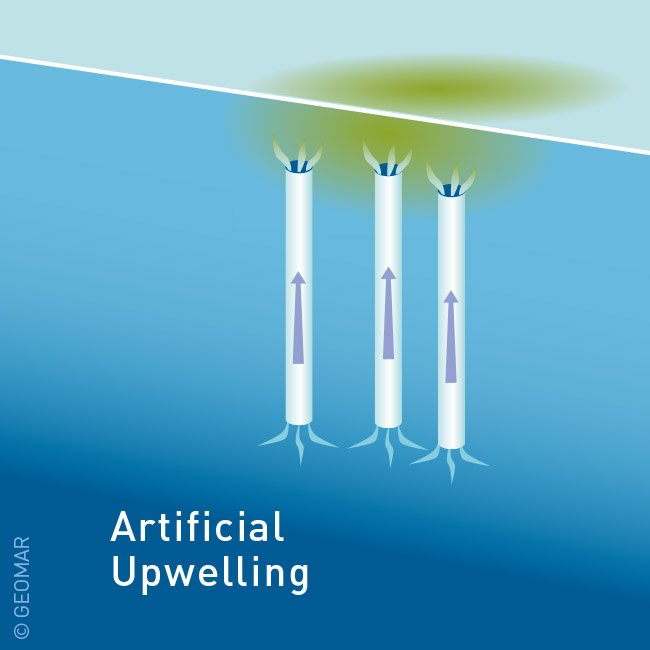
Deep Ocean Biomass Sinking
Plants on land and in the ocean pull in carbon dioxide from their environment during photosynthesis. The carbon contained in terrestrial or marine biomass, such as crops or macroalgae, could potentially be sequestered from the atmosphere for long periods of time by sinking it in the deep ocean.
Potential marine environmental impacts of this approach include:
- Smothering of organisms on the seafloor.
- Acidifying the deep ocean as the biomass is broken down.
- Altering nutrient balances in the deep ocean.
- Other impacts to sensitive deep ocean ecosystems.
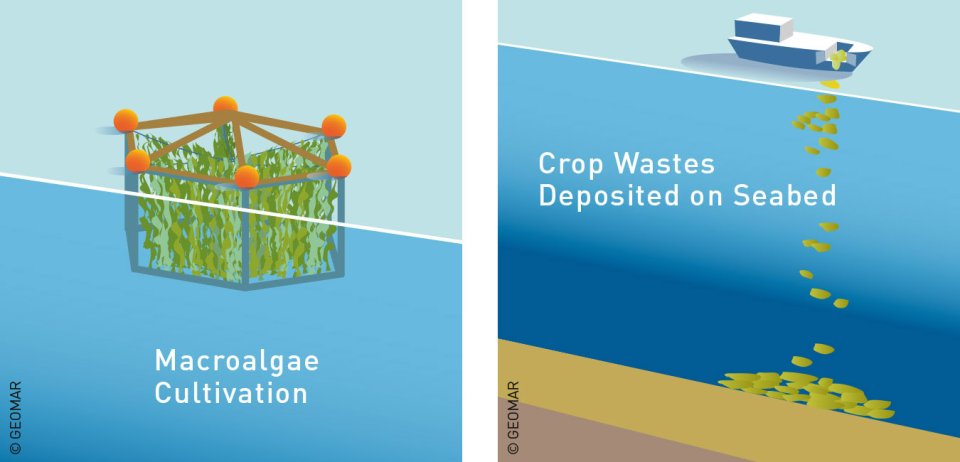